What is an Optical Lens?
An optical lens is a transparent optical component used to converge or diverge light emitted from a peripheral object. The transmitted light rays then form a real or virtual image of the object. A good example of transmissive optical components is lenses, meaning that pass or transmit light. Other transmissive components include filters, windows, flats, prisms, beamsplitters, and waveplates, while the opposite category — reflective (which reflects light rather than transmits it) — includes optical mirrors and retroreflectors. Lenses are the optical components that form the basic building blocks of many common optical devices, including cameras, binoculars, microscopes, and telescopes. Lenses are essentially light-controlling elements and so are exploited for light gathering and image formation.
A lens is typically made up of a transparent dielectric material like fused silica or optical glass with the front and back surfaces having a spherical curvature. Since the surfaces are curved, each ray of light that comes in parallel to the optical axis as shown in the figure below has a different value of θi to the surface normal. Each ray then refracts according to Snell's law. For a positive lens, this causes the light to converge toward its focal point on the right side of the lens while light will diverge from the focal point located on the left side of a negative lens. The significances of these operations are that lenses can be used for image formation as well as collection and collimation of light as shown in the figure below. The figures below illustrate how a lens affects incoming parallel light rays (left), applications of lenses (right) include creating a magnified image of an object (top), collimating light from a point source (middle), and focusing a collimated light source (bottom). There are several important aspects to optical imaging with lenses, including the relationship between object and image distances and the resulting magnification as well as the quality of the resulting images.
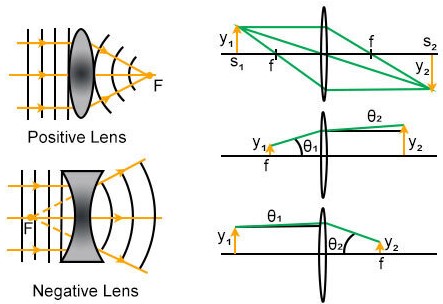
Advantages of lenses over mirrors
Curved mirrors and lenses can accomplish many of the same things in terms of light collection and image formation. However, lenses tend to be superior in terms of image formation because they are transparent, which allows light to be transmitted directly along the axis to the detector whereas mirrors require an off-axis geometry. Mirrors are typically preferred in terms of the light collection as they can be made significantly more lightweight than lenses and therefore can achieve larger diameters and light-collecting ability. This section discusses the mechanism of refraction that underlies the operation of a lens, issues that affect its performance, and the different lens types.
Refraction
Illustration of Snell's law of refraction at an interface between media of refractive indexes n1 and n2.
In addition to light reflecting off a planar interface between two media, it can also be transmitted and then refracted in the second medium as shown in the above figure. Refraction refers to the change in the angle of the incident light when it enters the second medium. Since the speed of light in a medium is inversely proportional to its index of refraction, it will either slow down or speed up when it enters a different medium, resulting in the light changing its direction. In the above figure, the refractive index of the second medium (n2) is greater than the first (n1), which results in a bending of the light toward the normal to the interface. This phenomenon of refraction is described by Snell's law:

where θi and θr are the angles of incidence and refraction, respectively. Snell's law enables the determination of the refracted ray direction provided the incident ray direction and refractive indices are known. This basic formula governs how lenses control the transmission of light for collection and imaging purposes.
Focal Length, Conjugate Ratio, and Lens Selection
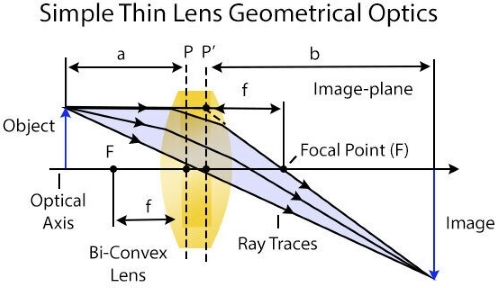
A lens's focal point is the point on the optical axis where light converges. Its focal length is the distance from the lens to this point, as indicated in the image. A positive lens has a positive focal length, while a negative lens has a focal length less than zero. The image below illustrates these two parameters.
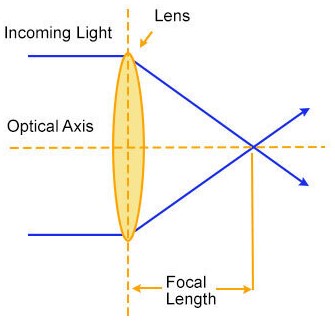
The conjugate ratio is defined as the ratio between the distance from the object's light source to the lens and the distance from the lens to the projected image. The endpoints of these two lengths are known as the object and image points. These two points lie on the lens's optical axis and are positioned so that light emitted from the object point will be focused at the image point. An object placed at the focal point of a lens results in an infinite conjugate ratio, while an object placed at twice the focal length results in an image formed at twice the focal length, giving a conjugate ratio of 1.
The images below illustrate the important optical points.
An application's conjugate ratio largely determines the ideal type of spherical lens. The table below shows the ideal lens shapes for an application's conjugate ratio.
Lens Shape | Ideal Conjugate Ratio |
Biconvex | <5:1 |
Plano-convex | All |
Plani-concave | Infinite, Larger finite (>5:1) |
Biconcave | <5:1 |
Meniscus | Varies; depends upon curvature and polarity |
Optical Aberrations and Types of Lenses
Ideal lenses would form perfect images or exact replicas of the object being imaged and would be able to focus collimated light to a spot size limited only by diffraction. However, real lenses are not perfect and induce optical aberrations, which cause degradation in the ability to form a high-quality image, collimate a beam, or focus it tightly. Monochromatic aberrations, i.e., no wavelength dependence, are common to both mirrors and lenses and come from the inability of spherical surfaces to focus light properly when it is far from the axis. These aberrations include spherical aberration, coma, and astigmatism. The figure below demonstrates the effects of spherical aberration (left) and chromatic aberration (right) on collimation when a point source is at the focal point. The impact of spherical aberration in a lens where rays with smaller angles are effectively collimated while rays with large angles converge instead. Unlike monochromatic aberrations, chromatic aberration only occurs in lenses. Due to the dispersion of the index of refraction of the lens material, different wavelengths will refract with different angles according to Snell's law. This causes degradation in image quality or light-gathering ability when broadband light is being used. Therefore selecting the correct lens type and polarity is heavily dependent upon the intended application, as described below. Choosing an ideal lens shape -- known as the application's best form -- is key to minimizing optical distortion and aberration.
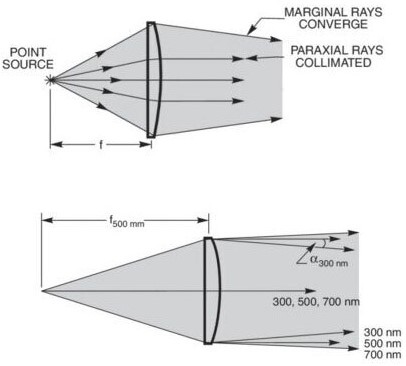
While spherical lenses do induce aberrations, choosing the proper lens shape can help minimize optical aberrations. For instance, plano-convex lenses, where only one side is curved, are the best choice for focusing parallel rays of light to a single point. Bi-convex lenses where both sides have a curvature that may not be equal are the best choice for imaging when the object and image are at similar distances from the lens. When a single spherical lens may be unsuitable due to spherical aberration, aspheric lenses may be used. These lenses have surfaces with tailored curvatures that help minimize the impact of aberrations but are typically expensive due to the complexities associated with fabrication. Alternatively, multiple spherical lenses can be used where one lens can cancel the aberration caused by another, as shown in the figure below. In addition to correcting for monochromatic aberrations, an achromatic doublet can be used to minimize chromatic aberrations by choosing the dispersion of the materials in the two lenses to produce a focal length that is independent of wavelength. Microscope objectives are multi-element lens systems that can significantly reduce the impacts of aberrations but are more expensive due to the complexity of the design. All the aforementioned lenses are rotationally symmetric, that is, light focuses the same regardless of which transverse axis it passes through. On the other hand, cylindrical lenses focus or expand light in one axis only and so are ideal for modifying an asymmetric beam like the output of a laser diode into a symmetric one. There are many features to take into consideration when choosing a lens, including focal length, lens shape, lens material, transmission properties, wavefront distortion, scattered light, types of coating, and cost.
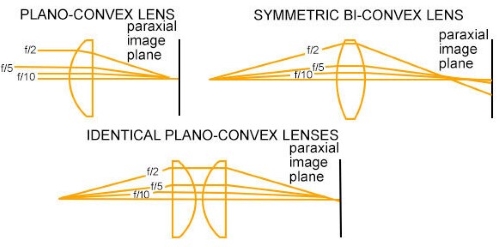
Shapes and Types
Lenses come in a variety of shapes including biconvex, biconcave, plano-convex, plano-concave, positive meniscus, and negative meniscus.
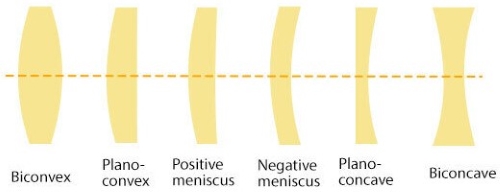
Lenses can be classified into two broad types: positive or converging and negative or diverging lenses.
Positive lenses cause a collimated beam of light by assuming the beam travels parallel to the lens axis and passes through the lens to converge or focus on a spot behind the lens. When referring to the image above, biconvex and plano-convex lenses are considered positive.
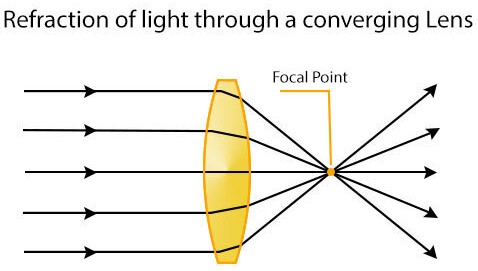
Negative lenses cause a collimated light beam to diverge and spread behind the lens. The two types of concave lenses — are biconcave and planoconcave.
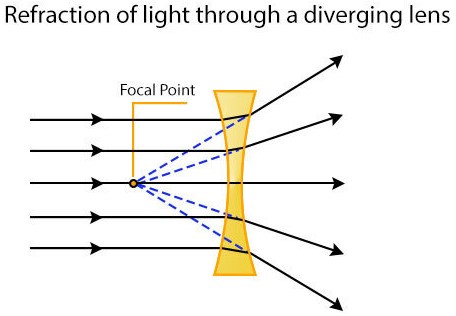
Meniscus lenses — a third broad type also referred to as convex-concave can be either positive or negative, depending on the curvature of both sides of the lens. A meniscus lens with a steep concave surface is negative, while a lens with a steeper convex surface will be positive. A meniscus lens with equal curvature on both sides would neither converge nor diverge light.
Spherical Lenses
Spherical lenses or singlets have curved surfaces that converge or diverge rays. All of the lens cross-sections are spherical lenses.
Cylindrical Lenses
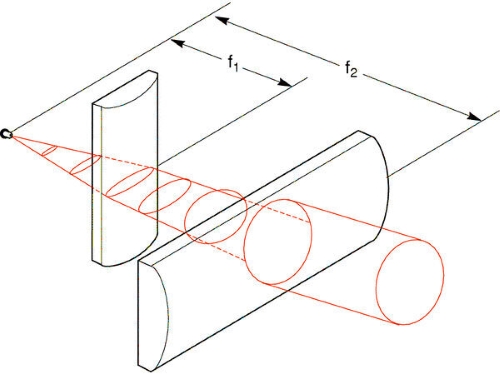
Unlike spherical lenses, cylindrical types have curved faces which can be considered part of a cylinder shape. This causes them to focus and transmit light to a line instead of a single point. Cylindrical lenses are commonly used to change an image's aspect ratio or shape a laser beam.
Achromats
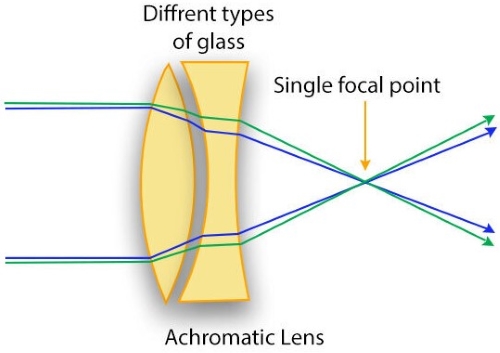
Achromats also known as achromatic lenses are used to minimize a special type of image distortion called chromatic aberration. This distortion occurs when a lens fails to focus all color wavelengths to the same convergence point, resulting in blurred contrast and color fringing. Achromats use at least two separate lens elements: one high-dispersion concave and one low-dispersion convex — to achieve their corrective effect. An achromatic doublet is shown in the figure above.
Fresnel Lenses
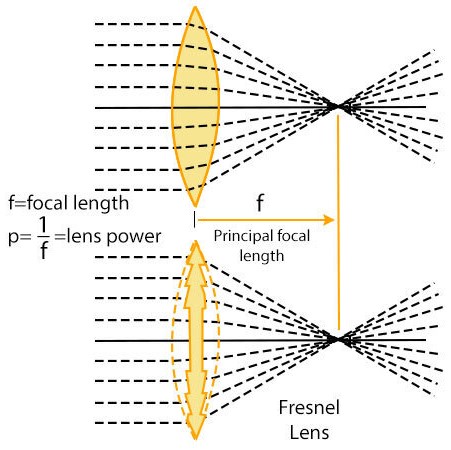
Fresnel lenses consist of thin, lightweight plastic sheets marked with a series of concentric grooves. Each groove serves as an individual refracting surface; the series of grooves bend collimated light to a common focal point. Fresnel lenses are a compromise between efficiency and optical quality: because the lens material is very thin, a very small amount of light is lost in the transmission process. A comparison between a standard biconvex lens and a Fresnel lens is shown in the figure above.
Gradient Index Lenses
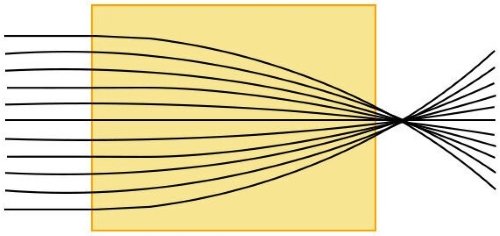
Gradient index (GRIN) lenses are simple planar lenses that continuously bend light rays within the lens until they finally converge on the focal point. This contrasts with conventional lenses, which primarily bend light abruptly when it exits the back of the lens material. GRIN lenses are therefore cost-effective and simple to employ. Additionally, the ability to precisely manufacture the length of the plane results in enormous flexibility to fit application parameters.
Specifications
Important specifications for optical lenses include wavelength and material.
Wavelength Range
After selecting the best lens type to fit their application, consumers should analyze the wavelength range of the application. When specifying a lens, manufacturers typically provide a range of electromagnetic radiation that the lens is designed to transmit. The wavelengths can be grouped into three broad groups: infrared, visible, and ultraviolet. A lens might not be limited to a single spectrum and may be able to transmit wavelengths from both the infrared and visible range, or visible and ultraviolet range, etc. Infrared lenses are designed to operate within the 750 to 2500 nm wavelength range. Lenses designed for use on the visible spectrum can transmit wavelengths within the 380 to 750 nm range. Ultraviolet lenses can transmit wavelengths between 4 and 380 nm.
Lens Material
Historically, optical lenses were constructed from transparent glass, but are now made from other materials like acrylics, polymers, and minerals. Lens material is determined by the raw material's dispersion and wavelength characteristics. For example, a lens designed for applications demanding low dispersion might be made of crown glass. Acrylic and polymer lenses are best suited to transmission within the visible spectrum, while minerals such as germanium and sapphire are suitable for a very wide range of wavelengths but particularly excel within the infrared spectrum.